The dependence of the main ionospheric trough shape on longitude, altitude, season, local time, and solar and magnetic activity
A. T. Karpachev
Institute of Terrestrial Magnetism, Ionosphere, and Radiowave Propagation, Russian Academy of Sciences, Troitsk, Moscow region, 142190 Russia
e-mail: karp@izmiran.ru
Pages:
1 |
2 |
3 |
4 |
5
Variations in the main ionospheric trough (MIT) shape are studied based on Intercosmos-19,
Cosmos-1809, and Cosmos-900 satellite data. The main dependences are revealed and the causes
of the strong differences between results of different studies are analyzed. In particular,
it has been shown that an analysis of the MIT shape variations without taking into account
the corresponding longitude and hemisphere leads to contradictions. It is also shown that the
problem of the MIT shape variations with increasing magnetic activity cannot be solved merely
by a data averaging. This problem becomes especially difficult at low solar activity. The MIT
shape variations are governed by the longitudinal-latitudinal variations in the electron
concentration in the trough region, in turn, dependent on several factors. The influence of
the main factors, such as the illumination of the high-latitude ionosphere, neutral wind,
electric field of the magnetospheric convection, and auroral particle precipitation, is
qualitatively discussed.
1. INTRODUCTION
The MIT shape depends on many factors including altitude, longitude, local
time, season, and solar and geomagnetic activity. The following example can demonstrate the
degree of the dependence: a deep and narrow trough is always observed in summer night-time
conditions at a solar activity minimum, whereas during a solar activity maximum a weakly
pronounced trough is formed only at nonsunlit longitudes or at high magnetic activity
[Besprozvannaya, 1975; Karpachev, 1995, 1996]. Though fairly much attention has been paid to
MIT shape studies, the majority of the questions related to MIT shape are yet obscure and
contradictory. For example, Ben'kova et al. [1993] claim that the trough is most developed at
heights of the F2 layer maximum. The vertical cross section of the topside ionosphere obtained
by Mendillo and Chacko [1977] demonstrates that the depth of the trough does not change at
heights of 450-950 km. However, according to Legen'ka [1974], the trough depth increases to
heights of 800-1000 km. One third of the publications states that MIT becomes narrower and
deeper with increasing magnetic activity. In the second third, it has been found that the MIT
becomes less pronounced. In the rest of the publications, no pronounced dependence was
detected. Based on Intercosmos-19 data for only one longitudinal sector
(20-70
oE) in the
Northern Hemisphere, Ben'kova et al. [1988] revealed at least four types of troughs strongly
different in their shape. In the relatively old publication, this situation was assessed in the
following way: "An analysis of diurnal, seasonal, and altitude variations in the trough
characteristics leads to a confusion" [Ahmed et al., 1979]. The situation has changed slightly
since then. Meanwhile, the ignorance of the MIT shape variations hinders the solution of many
problems. In particular, this leads to considerable uncertainties in solving trajectory
problems for radiowave propagation across polar regions of the ionosphere [Blagoveshchensky
and Zherebtsov, 1987]. Therefore, radiophysicists should use in calculations a crude
approximation made in the Halcrow and Nisbet [1977] model or determine trough parameters
using the HF-network signals [Blagoveshchensky and Borisova, 1995]. The MIT characteristics
(width, depth, height and wall steepness) strongly affect the propagation (guiding) of
different natural and artificial signals [Kleimenova et al., 1995, Blagoveshchensky et al.,
1997; Clilverd et al., 1995]. The aforesaid has specified the goal of this paper: based on a
large data array, to study the MIT shape dependence on different factors, to establish the
main regularities, and to understand the causes of contradictions between different studies
in order to make progress in solving the problems stated.
There is no commonly used MIT definition [Galperin et al., 1990]. A point of inflection in
the latitudinal profile of Ne at the MIT equatorial wall is used to determine the presence of
MIT. If a background decrease in Ne towards high latitudes (related mainly to the decrease in
the solar zenith angle and in the O/N
2 ratio) replaces by a more steep decrease caused by an
additional factor, then a deep minimum of Ne formed at subauroral latitudes is defined as
a trough. According to this definition of MIT, its width and depth are counted from an
inflection point at Ne latitudinal profile. For example, in Fig. 1c the inflection point is
located at invariant latitudes (

) of 52-54
o. In reality, the location
of an inflection point
and, consequently, the discussed MIT characteristics are often determined rather uncertainly
(e.g., see Fig. 1a). To avoid the uncertainty, the MIT width is determined at levels of
1.5N
min or 2N
min. However,
this method may lead to even larger uncertainties in the MIT
width. The MIT depth is sometimes determined relative to the MIT polar wall which is as
a rule more pronounced than the equatorial wall at heights of the F2 layer. However, the
spread in data at the polar wall is very considerable and so the depth becomes widely variable.
Therefore, we will consider that the first method is physically most adequate in spite of its
imperfection.
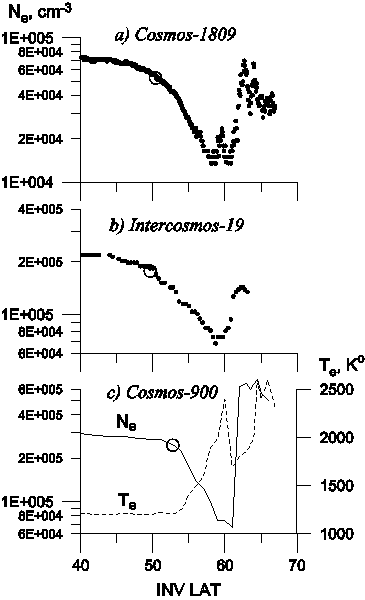
Fig. 1.
|
Examples of observations of the MIT onboard
(a) Cosmos-1809 (January 10, 1989, 1800 LT, Kp = 3-, h = 1000 km);
(b) Intercosmos-19 (September 30, 1980, 0430 LT, Kp = 20, h = 600 km); and
(c) Cosmos-900 (July 26, 1979, 0055 LT, Kp = 2-, 375 km).
The circle marks the approximate position of the inflection point at the MIT equatorial wall.
|
2. DATA
The large data set of Intercosmos-19 (~300 orbits), Cosmos-1809 (~100 orbits), and Cosmos-900
(~1200 orbits) data is used to analyze MIT characteristics. Data have been obtained both for
high (F
10.7 ~ 200, 1979-1980) and
low (F
10.7 ~ 70, 1986-1987) solar activity. They cover all
longitudes in both hemispheres, the 1800-0600 LT time interval, and the magnetic activity
range Kp = 0-8. Topside sounding data onboard the Intercosmos-19 and Cosmos-1809 satellites
can be obtained within the entire range from the height of the F2 layer maximum to the
height of the satellite. The Intercosmos-19 satellite had got an elliptical orbit with an
altitude varying from 500 to 1000 km. As an example, the MIT registered by Intercosmos-19
at a height of 600 km at 0430 LT on September 30, 1980 (Kp = 2o) in the Northern Hemisphere
is shown in Fig. 1b. The MIT polar wall is less pronounced then the equatorial wall; however,
the trough is fairly deep and is easily observed. The Cosmos-1809 satellite had an almost
circular orbit with a height of about 1000 km. Figure 1a shows the MIT registered onboard
Cosmos-1809 at 1800 LT on January 10, 1989 (Kp = 3-) at a height of 1000 km in the Northern
Hemisphere. Both walls of the trough are equally pronounced. The trough bottom has a
complicated structure caused by the prehistory, which will also be discussed below. The
Cosmos-900 satellite had a circular orbit. Its altitude decreased during the observations
from ~500 to ~350 km. Figure 1c shows MIT registered by Cosmos-900 at 0055 LT on July 26,
1979 (Kp = 2-) at a height of about 375 km in the Southern Hemisphere, i.e., almost at a
maximum of the nighttime F2 layer. A peak of Te usually accompanies a trough in Ne [Smilauer
and Afonin, 1983]. As a rule, more pronounced troughs correspond to more distinct peaks in
Te (e.g., see Fig. 1c). A Te peak is observed at latitudes of the trough bottom, but a Te
maximum not always coincide with an Ne minimum (see also Fig. 1c).
3. MIT SHAPE LONGITUDINAL VARIATIONS UNDER NIGHTTIME WINTER
CONDITIONS
The location of a trough minimum can vary with longitude by 10-11 at different latitudes,
and the electron concentration can change by an order of magnitude [Deminov and
Karpachev, 1986a]. This leads to considerable variations in the trough shape with
longitude. An ignorance of this fact often leads to contradictions. Therefore, we should start
analyzing the MIT shape variation from the longitudinal effect (LE). The trough shape
dependence on longitude (even based on satellite data) was traditionally analyzed by
comparing data for two or three longitudinal sectors [Ben'kova and Zikrach, 1983;
Besprozvannaya, 1975; Besprozvannaya and Makarova, 1984; Tulunay, 1973]. However, it is
impossible to determine the LE character and, especially, to understand causes of its
formation on the basis of two-three points. We analyze the MIT shape variations with
longitude on the basis of the global (for all longitudes) distribution of NmF2 in the MIT
region. Figure 2 shows the longitudinal variations in NmF2 at fixed invariant latitudes and
at a trough minimum and in the location of a trough minimum in the Northern and Southern
hemispheres. The variations are revealed by averaging the data array obtained onboard the
Intercosmos-19 satellite for the near-midnight (0000 - 0200 LT) winter (December-January)
quiet (Kp <= 3) conditions at high solar activity
(F
10.7 ~ 200). A striking feature is a
strong asymmetry in the hemispheres both in the position of a trough minimum and in the
electron concentration in the entire trough region. The amplitude of the NmF2 longitudinal
variation at a trough minimum in the Northern Hemisphere is
A = N
max/N
min ~ 4. At
the same time, the minimum (0.9x10
5
cm
-3) and maximum (3.4x10
5
cm
-3) of NmF2 are reached at longitudes of
190-200
o and 300
o E,
respectively.
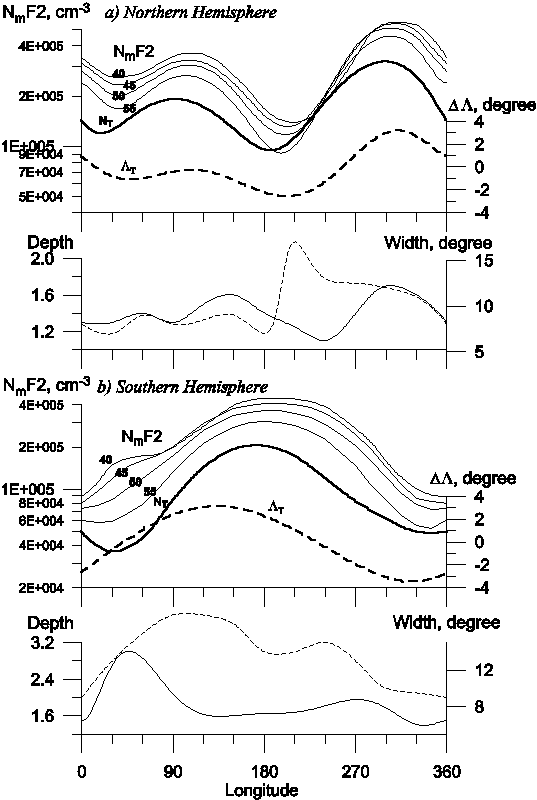
Fig. 2.
|
Longitudinal variations in NmF2 at invariant latitudes of 40, 45, 50, and
55o (thin curves) and at a MIT minimum
(NT, thick curve), the position of a MIT minimum (LT, thick dashed curve), and the MIT
depth (solid curve) and width (dashed curve) according to Intercosmos-19 data for quiet
winter near-midnight conditions in (a) the Northern and (b) the Southern hemispheres.
|
The amplitude of the NmF2 longitudinal variations in the Southern Hemisphere is larger then
in the Northern Hemisphere and reaches ~5.5 at a trough minimum. At the same time, Fig.
2b shows that the minimum
(3.7x10
4
cm
-3) and maximum
(2.1x10
5
cm
-3) of NmF2 are observed at longitudes of
30
o and 170-180
o, respectively.
The amplitude of the longitudinal effect on the trough location is also larger in the
Southern Hemisphere then in the Northern Hemisphere (6
o and
5
o, respectively).
The causes of LE on the trough location and form were qualitatively analyzed by
Deminov and Karpachev [1986a] and Deminov and Karpachev [1986b], respectively. The causes
of the NmF2 and hmF2 longitudinal variations in the trough region will be studied
in detail on the basis of calculations in the next paper. So, omitting the causes
of LE, we note here the main points. The position of a trough minimum and the plasma
concentration in the trough are widely variable with longitude. The Ne longitudinal
variations in the mid-latitude Northern Hemisphere are similar to the Ne variations
at a trough minimum but differ in their phase and amplitude. In the Southern Hemisphere
they are also considerably different in form. As a result, the trough shape varies with
longitude.
Next page »